If you are looking for a Bachelor, Master, or PhD position, please have a look at the different research topics we are offering at the moment.
PhD- and Postdocs-Positions
In cooperation with the Qnami AG, Switzerland we can offer a
PhD position in Quantum Sensing (m/w/d)
Bachelor- and Master-Positions
We are currently seeking a dedicated Master's or PhD student to join our research team. Our project is centered on the growth and characterization of high-performance ensemble NV center diamonds, a key direction in the fields of quantum computing and quantum sensing. This unique opportunity allows you to explore the intriguing realm of quantum physics and contribute to the advancement of state-of-the-art quantum technologies.
Our state-of-the-art infrastructure includes:
- CVD diamond growth equipment.
- Ion implantation equipment.
- Heat treatment equipment.
- A clean room with a complete set of micro-nano processing capabilities.
Key Responsibilities:
- Conducting innovative research on the CVD growth and NV doping as well as characterization of high-performance ensemble NV center diamonds.
- Developing and applying quantum sensing techniques using these NV doped diamonds as a platform.
- Collaborating with a multidisciplinary team to enhance our understanding of quantum phenomena in diamond materials.
- Participating in related quantum applications, including high-precision magnetic field measurements and research on quantum many-body problems.
Qualifications:
- Enrollment in a Master's or PhD program in Physics, Material Science, or a related field.
- A solid foundation in quantum physics and material science.
- Exceptional problem-solving skills and a passion for scientific exploration.
Contact: Jixing Zhang/Andrej Denisenko
References:
Dieter Suter, Fedor Jelezko, Single-spin magnetic resonance in the nitrogen-vacancy center of diamond, Progress in Nuclear Magnetic Resonance Spectroscopy, Volumes 98–99, 2017, Pages 50-62, ISSN 0079-6565, https://doi.org/10.1016/j.pnmrs.2016.12.001.
Atatüre, M., Englund, D., Vamivakas, N. et al. Material platforms for spin-based photonic quantum technologies. Nature Reviews Materials 3, 38–51 (2018). https://doi.org/10.1038/s41578-018-0008-9
Bachelor- and Master-Positions | physics and photonic engineering
at the Zentrum für Angewandte Quantentechnologie / 3. Physikalisches Institut
We are now offering a master or bachelor thesis for physicists and photonics engineers.
Our group develops diamond-based magnetic field sensors with a sensitivity that is 1 million to 1 billion times finer than the earth's magnetic field. In addition to the goal of implementing quantum sensor technology in sophisticated medical applications such as magnetomyography (MMG), there is a wide range of industrial applications.
This goal poses great challenges for us, among others in the fields of optics (mesoscopic and macroscopic) and microwave technology. Here we cooperate with different institutes, e.g. IIS, PI4, ITO, IFM.
For development we use software like Zemax, Ansys, Inventor, Python, Matlab and technologies like AFM, Confocal microscopy, Nanoscribe 3D-microfabrication, Ion beam etching, Sputtering, micromanipulator, CVD & HPHT diamond processing. Besides, we have activities such as sensor setup, spin manipulation (cw/pulsed protocols), software/hardware development, signal analysis.
Through increasing experience and further development of the techniques, the sensitivity can be further improved and the sensor can be made compact and robust against environmental influences.
You are motivated, can work independently, bring basic knowledge in physics, photonics, (microwave technology), programming languages ... we are looking forward to your visit at ZAQuant.
The task of the project would be to perform some NMR pulsed protocols. Benchmark all-optical readout of the nuclear spin ensemble. Develop new NMR protocols for sensing.
A good candidate should be motivated to work in collaboration with Engineering department on modern CMOS EPR-on-chip platforms. A strong programming skill as well as background of spin physics (EPR, NMR) is required.
Contact / supervisor: PhD Vadim Vorobyov
Related references:
[1] Soshenko, V.V., Bolshedvorskii, S.V., Rubinas, O., Sorokin, V.N., Smolyaninov, A.N., Vorobyov, V.V. and Akimov, A.V., 2020. Nuclear spin gyroscope based on the NV center in diamond. arXiv preprint arXiv:2009.00916 https://arxiv.org/pdf/2009.00916.pdf
[2] Jarmola, A., Fescenko, I., Acosta, V.M., Doherty, M.W., Fatemi, F.K., Ivanov, T., Budker, D. and Malinovsky, V.S., 2020. Robust optical readout and characterization of nuclear spin transitions in nitrogen-vacancy ensembles in diamond. Physical Review Research, 2(2), p.023094.
A good candidate is familiar with programming with python and quantum mechanics as well as QISKIT environment.
References:
[1] QISKIT
[2] Waldherr, G., Wang, Y., Zaiser, S. et al. Quantum error correction in a solid-state hybrid spin register. Nature 506, 204–207 (2014). https://doi.org/10.1038/nature12919
Contact / supervisor: PhD Vadim Vorobyov
A good candidate is familiar with programming with python and quantum mechanics as well as QISKIT environment.
References:
[1] QISKIT
[2] Johannes Jakob Meyer, Johannes Borregaard, Jens Eisert A variational toolbox for quantum multi-parameter estimation https://arxiv.org/abs/2006.06303
Contact / supervisor: PhD Vadim Vorobyov
A good candidate is familiar with programming with python or schema programming language. CUDA GPU experience is appreciated.
Content:
- An FDTD algorithm for numerical simulation of electromagnetic fields
- Optimisation strategies:
- Gradient ascent, Stochastic methods, machine learning, inverse deig - Collaboration with experimentalist and close loop feedback production – simulation cycle.
References:
[1] http://ab-initio.mit.edu/book/
[2] Qimin Quan and Marko Loncar, "Deterministic design of wavelength scale, ultra-high Q photonic crystal nanobeam cavities," Opt. Express 19, 18529-18542 (2011) link
Contact / supervisor: PhD Vadim Vorobyov
Lithium niobate is an electro-optic material whose refractive index changes upon application of an external electric field. At the same time, it can be produced in a form of thin film and subsequently shaped into on-chip photonic elements. These elements can be manipulated by electrically routing light within the photonic circuits of arbitrary complexity. Lithium niobate can also be activated with rare-earth ion by ion implantation to serve as a source of single photons and, at the same time, to serve as a quantum memory thanks to their electron and nuclear spins. Our particular interest is 171Yb isotope. Its hyperfine structure in lithium niobate is vaguely known. The main point of the proposed bachelor project is to reveal the hyperfine splittings of the ground and of the excited optical states by means of optical hole-burning and optically detected magnetic resonance techniques.
Contact / supervisor: PhD Roman Kolesov
Modern quantum information processing relies on fast and precise control of quantum bits. Algorithms require the fidelity of each operation to exceed 99% and indeed scalable quantum computers require even larger numbers. Achieving such high precision requires sophisticated quantum control. In the thesis we will apply quantum optimal control algorithms to single quantum bits. This numerical method achieves high gate fidelities by optimizing quantum control under a predefined stet of boundary conditions. We will install these algorithms on new ultrafast hardware with the goal to attain increased precision in quantum error correction. The achieved gain in fidelity will be experimentally tested and the developed software will be integrated into an existing software package.
Contact: Prof. Dr. Jörg Wrachtrup
Rare earth ions in optical crystals are potentially excellent candidates for quantum computing applications as qubits and quantum memory. So far, most of quantum features of rare earth ions were studied and exploited in ensembles containing many (billions) of ions. However, for scalable quantum computing single ion qubits are preferred. As of now, only two rare earth species, namely, cerium and praseodymium, were detected optically at a single ion level. Extending the list of detectable ions would strengthen the whole field of rare earth quantum computing.
The proposed master project is aiming at optical detection of a single divalent europium ion in various hosts. While some of the host materials, such as CaF2, are readily available as single crystals, the most interesting having no intrinsic nuclear spins ones can be obtained primarily as micro- and nano-powders. If the detection of Eu2+ ion is achieved at early stage of the project, the focus of the work will be shifted on studying electron and nuclear spin properties of the potential europium qubit.
The project will involve many aspects of materials science and optical characterization in confocal microscopes. It also implies close collaboration with material science groups at Max-Planck Institute as well as with implantation groups in Bochum, Augsburg, and Leipzig.
Interested applicants can contact Prof. Dr. Jörg Wrachtrup or Dr. Roman Kolesov by e-mails
Contact
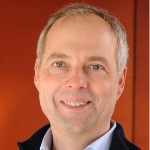
Jörg Wrachtrup
Prof. Dr.Head of Department
[Photo: David Ausserhofer]